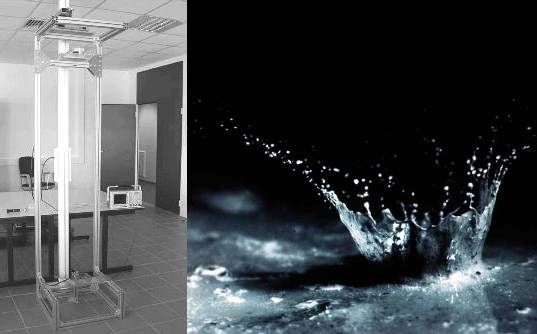
The testing apparatus controlled drop size, frequency, and height, as drops of water fell and impacted a piezoelectric material at the base. Credit: Romain Guigon, et al.
Researchers who study energy harvesting see energy all around us – we just need to find a way to capture that energy. One of the latest energy harvesting techniques is converting the mechanical energy from falling raindrops into electricity that can be used to power sensors and other electronics devices.
Scientists from CEA/Leti-Minatec, an R&D institute in Grenoble, France, specializing in microelectronics, have recently developed a system that recovers the vibration energy from a piezoelectric structure impacted by a falling raindrop. The system works with raindrops ranging in diameter from 1 to 5 mm, and simulations show that it’s possible to recover up to 12 milliwatts from one of the larger “downpour” drops.
“Our work could be considered as a good alternative to power systems in raining outdoor environments where solar energy is difficult to exploit,” Thomas Jager told PhysOrg.com. He explained that the system could be used for both mobile outdoor devices as well as indoor power. “For example, we intend to develop remote sensor nodes in cooling towers, but abandoned sensor networks are also one of the foreseen applications for this type of system.”
As Jager and coauthors Romain Guigon, Jean-Jacques Chaillout, and Ghislain Despesse explain in a recent issue of Smart Materials and Structures, the physics of how a raindrop impacts a surface is not fully understood. However, to build a rain energy harvesting system, the important part is to estimate the recoverable energy during the impact.
When a raindrop impacts a surface, it produces a perfectly inelastic shock. The amount of energy generated by the impact can then be estimated using a mechanical-electric model.
To capture the raindrops’ mechanical energy, the scientists used a PVDF (polyvinylidene fluoride) polymer, a piezoelectric material that converts mechanical energy into electrical energy. When a raindrop impacts the 25-micrometer-thick PVDF, the polymer starts to vibrate. Electrodes embedded in the PVDF are used to recover the electrical charges generated by the vibrations.
The group experimented with raindrops of different sizes, falling heights, and speeds. They found that slow falling raindrops generate the most energy because raindrops falling at high speeds often lose some energy due to splash. By using a micropump to generate and test the properties of raindrops, the researchers demonstrated that, for low drop heights, the electrical energy is proportional to the square of the drop’s mechanical energy, while voltage and mechanical energy are directly proportional.
The largest raindrops caused the largest vibrations on the PVDF, and therefore generated the greatest amount of electrical energy. The researchers demonstrated that their system could generate 1 microwatt of continuous power as a worst-case scenario, while simulations showed that a single large raindrop might generate up to 12 milliwatts of power.
“The recoverable energy depends directly on the size of the piezoelectric membrane, the size of raindrops, and their frequency,” Jager explained. “The available energy per drop varies between 2 µJ from 1 mJ depending on its size.
“The corresponding instantaneous converted power starts from a few µW up to 10 mW for a converter area of a several square centimeters. An interesting figure to keep in mind could also be the available rain power per year in common France regions with a continental climate: almost 1 Wh per square meter per year.”
In the future, the scientists plan to develop a method to store the electrical power to provide a steady current for practical use.
More information: Guigon, Romain, Chaillout, Jean-Jacques, Jager, Thomas, and Despesse Ghislain. “Harvesting raindrop energy: theory” and “Harvesting raindrop energy: experimental study.” Smart Mater. Struct. 17 (2008) 015038-9.
Copyright 2008 PhysOrg.com.
All rights reserved. This material may not be published, broadcast, rewritten or redistributed in whole or part without the express written permission of PhysOrg.com.